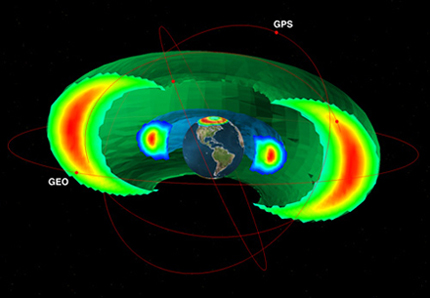
Space Weather in Earth's Radiation Belts
The Earth's Radiation Belts are regions of near-Earth space that contain high energy electrons and protons. The Inner Belt contains both high-energy electrons and protons, whereas the Outer Belt (or Belts) are dominated by high-energy electrons that are trapped in the geomagnetic field . James van Allen and his team made the unexpected discovery of the Radiation Belts at the beginning of the Space Age , and as our space age progresses, it is clear that some of the most useful orbits (see Figure 1) lie directly inside the Belts. The "weather" of the Radiation Belts is therefore a very important consideration for satellite design, orbit planning and the construction of space-based technology. High-energy electrons can damage sensitive satellite components by causing electrostatic discharges either on the surface or deep inside the spacecraft.
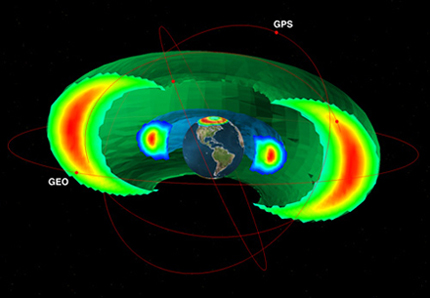
The study of Space Weather in the Radiation Belts is an attempt to describe the variation of the location and strength of the cloud of high-energy electrons that form the most dangerous part of the Radiation Belt. We seek to describe the range of possible radiation environments, as well as understand what can make the amount and energy of high-energy electrons change. Data from orbiting spacecraft indicate that in response to geomagnetic storms, the amount of high-energy electrons can (A) increase, (B) decrease or (C) stay the same. Additionally, the high-energy electron population can also move inwards or outwards relative to the Earth, bring them into contact with different orbits, and bringing some spacecraft into and out of danger.
What causes the variability in the Outer Radiation Belt?
In a nutshell, the Solar Wind. The solar wind is the name for the ionized gas that streams out from the solar surface into the solar system. It speeds past the Earth at an average speed of around 400 km/s, although its speed can vary enormously. The solar wind is very tenuous, with only about ten proton/electron pairs every cubic centimetre, but the density can vary rapidly by up to a factor of ten. The charged solar wind also carries with it the magnetic field from the sun, and this magnetic field can vary in strength and orientation. The Earth's magnetosphere protects us, for the most part, from the solar wind, but the variability of the plasma flow and magnetic field direction are the driving forces of Space Weather.
Electrons have a very small mass and so the gravitational force on a Radiation Belt electron is very weak. Since electrons are charged particles, they are controlled instead by the Earth's magnetic field, and other electromagnetic fields in near-Earth space. The shape of the inner and outer Radiation Belts is entirely due to the geomagnetic field (see Figure 1). Electrons are trapped on paths that gyrate around the magnetic field direction, and bounce between hemispheres (see Figure 2), guided at all times by the shape of the Earth's magnetic field (grey lines).
Scientific research over the last few decades suggests that the amount and energy of electrons in the Outer Radiation Belt is controlled by a delicate balance between multiple processes:
- Magnetospheric topology: The shape of the magnetosphere determines where electrons can be trapped along bouncing paths. In turn, the ram pressure and magnetic field orientation of the solar wind determines the shape of the magnetospheric cavity. Typically, if electron paths are pushed closer to the Earth, then the electrons become energized due to conservation of the magnetic moment of the particle.
- Large-scale electric fields: A large-scale dawn-dusk convection electric field is induced inside the magnetosphere by the flow of the solar wind past the Earth. More transient and localized electric fields are induced during the rapid reconfiguration of the night-side magnetic field known as a substorm.
- Ultra-low frequency waves: The magnetospheric cavity supports electromagnetic waves at ultra-low frequencies (ULF) that take the form of a global "ringing" of Earth's magnetosphere.The ULF waves slowly transport high-energy particles in the radial direction towards or away from the Earth and drive particles into the upper atmosphere.
- Locally-unstable electromagnetic waves: Regions of free energy in the plasma such as temperature anisotropy promote the localized growth of electromagnetic waves. These waves act to diffuse the free energy through pitch-angle scattering (changing the particle direction), or energy diffusion (changing the particle speed). A small fraction of electrons can be resonantly accelerated to very high energies.
Sources and Sinks
The Outer Radiation Belt is not an isolated system; it is an integral part of the magnetosphere and only one facet of the entire Solar-Terrestrial Interaction. The physical processes listed above describe the mechanisms that can accelerate and move high-energy plasma around the magnetosphere, but it is important to remember the sources and sinks of plasma in the Outer Radiation Belt.
- Source - substorm injections: One of the by-products of a magnetospheric substorm is the injection of high-energy plasma in the region around midnight. These high energy protons and electrons drift around the Earth in different directions according to their charge (electrons drift dawnwards from midnight and protons drift duskwards).
- Sink - energetic particle precipitation: As electrons bounce from hemisphere to hemisphere, the height at which they "bounce" can vary, either due to changes in the shape of the geomagnetic field, or due to interactions with electromagnetic waves (see Process III or Process IV). If the bounce height (often known as a mirror point - see Figure 2) reaches into the upper atmosphere, there is a high chance that the electron will collide with a neutral atom or molecule and be lost from the magnetosphere. This process is known as particle precipitation and can result in the Aurora and in changes in the ionization of the upper atmosphere.
- Sink - magnetopause shadowing: As electrons drift around the Earth, their paths may intersect the magnetopause. In this case, the electrons are lost into the solar wind.
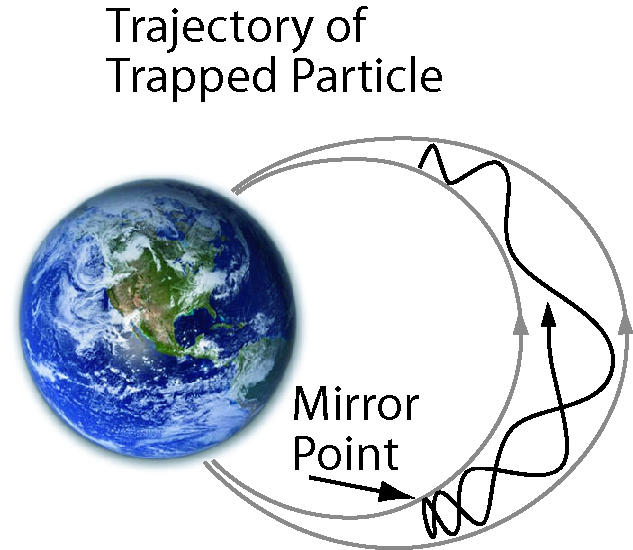
Radiation Belt Research in the Department of Meteorology
Dr Clare Watt is working on a new numerical model that will include all processes discussed above, as well as the sources and sinks of plasma in the Outer Radiation Belt.
Published research includes:
- A new numerical model to study locally-unstable electromagnetic waves (Process IV) called WAGER. The model uses geometric optics (also known as raytracing), and the equation of radiation transfer to build wave spectra that take into account the propagation paths of electromagnetic waves, as well as the local plasma conditions in order to predict wave characteristics due to temperature anisotropy.
- A study of variations in the growth rates of locally-unstable whistler-mode waves when the whole system is slowly modulated by the presence of an ultra-low frequency wave (or, what happens when Process III and Process IV happen at the same time!)