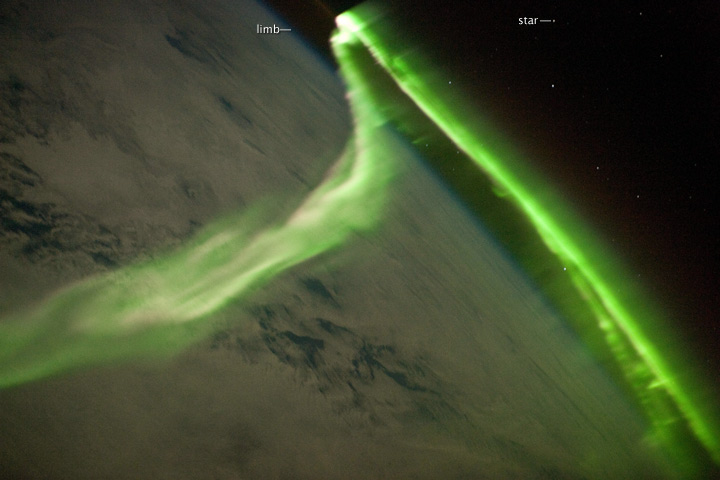
Earth's Aurora
The Aurora Borealis and Australis (see Figure 1) are a beautiful manifestation of the Solar-Terrestrial Interaction. They result when magnetospheric electrons and protons with energies greater than around 1keV collide with neutral atoms and molecules in the upper atmosphere. The atoms are excited to a higher energy state during the collision, and in order to lose their excess energy, they emit light.
Although we have long known why light is emitted in the upper atmosphere during an auroral display, we have much less information about why aurora appear to be organized in long thin strips like the aurora in Figure 1. These strips, known as auroral arcs, are sometimes fairly static, but sometimes highly dynamic.
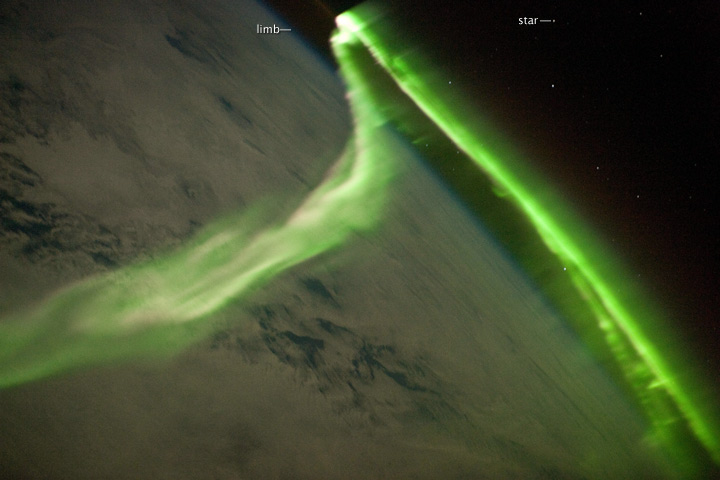
Auroral Acceleration
Typically, to create bright auroral arcs, electrons must be accelerated down towards the Earth from space. This acceleration happens in the direction parallel or antiparallel to the geomagnetic field. It is now generally accepted that there are two different types of acceleration:
Quasistatic potential drops: On time scales much longer than the time it takes for an electron to bounce between mirror points, parallel electric fields develop at low altitudes just above the Earth's ionosphere. There is a great deal of observational evidence for this type of acceleration mechanism from in-situ spacecraft measurements.
In spacecraft measurements, the differential electron energy flux is enhanced over a very narrow range of energies as if they have all experienced the same accelerating electric field - i.e., this behaviour suggests that the electric field structure is essentially static.
Acceleration in Shear Alfvén waves: sometimes referred to as Alfvenic acceleration. The magnetosphere supports low-frequency electromagnetic waves that carry energy and information over vast distances. One type of low-frequency waves - Shear Alfvén waves - are transverse oscillations of the magnetic field that can support parallel electric fields when their perpendicular wavelengths are small. Under these circumstances, electrons can gain sufficient energy to create the aurora.
Auroral research in the Department of Meteorology
Numerical Models: Dr Clare Watt has created a novel simulation model to study the acceleration of electrons in Shear Alfvén waves. The simulation model, DK1D1, reproduces many observed features. For example,
At low altitudes, DK1D demonstrates acceleration over a broad range of energies, and mirroring electrons producing horseshoe shaped distributions. (see Figure 2 below). The simulation shows that some of these low-energy electrons are merely oscillating about a fixed location in space, and experience little net acceleration towards the ionosphere. At low altitudes, the electrons mirror in the converging geomagnetic field, forming the distinctive "horseshoe" shaped distributions. These distributions can be unstable to Auroral Kilometric Radiation.
At high altitudes, DK1D reproduces the parallel energisation observed by the Polar spacecraft. Due to the nonlinear trapping of electrons in the shear Alfvén wave (see Figure 3 below), electrons appear to be energized in the direction parallel and anti-parallel to the field. An important characteristic of this energisation is that only low-energy (<1keV) electrons display parallel energisation; the higher energy electrons are essentially isotropic (see Figure 4 below). This behaviour clearly demonstrates that electrons interact with the wave in different ways depending upon their velocity.
In standing shear Alfvén waves, DK1D demonstrates that acceleration of electrons is efficient in both cold and warm plasma.
Observational Evidence: In collaboration with a team led by Dr Colin Forsyth and Prof Andrew Fazakerley at the Mullard Space Science Laboratory, Dr Watt is helping to research the structure and slow evolution of quasistatic potential drops using observations from the Cluster spacecraft.
Auroral Science Reviews: Dr Watt presented two reviews recently at the International Association of Geomagnetism and Aeronomy (IAGA) General Assemblies. They can be found here
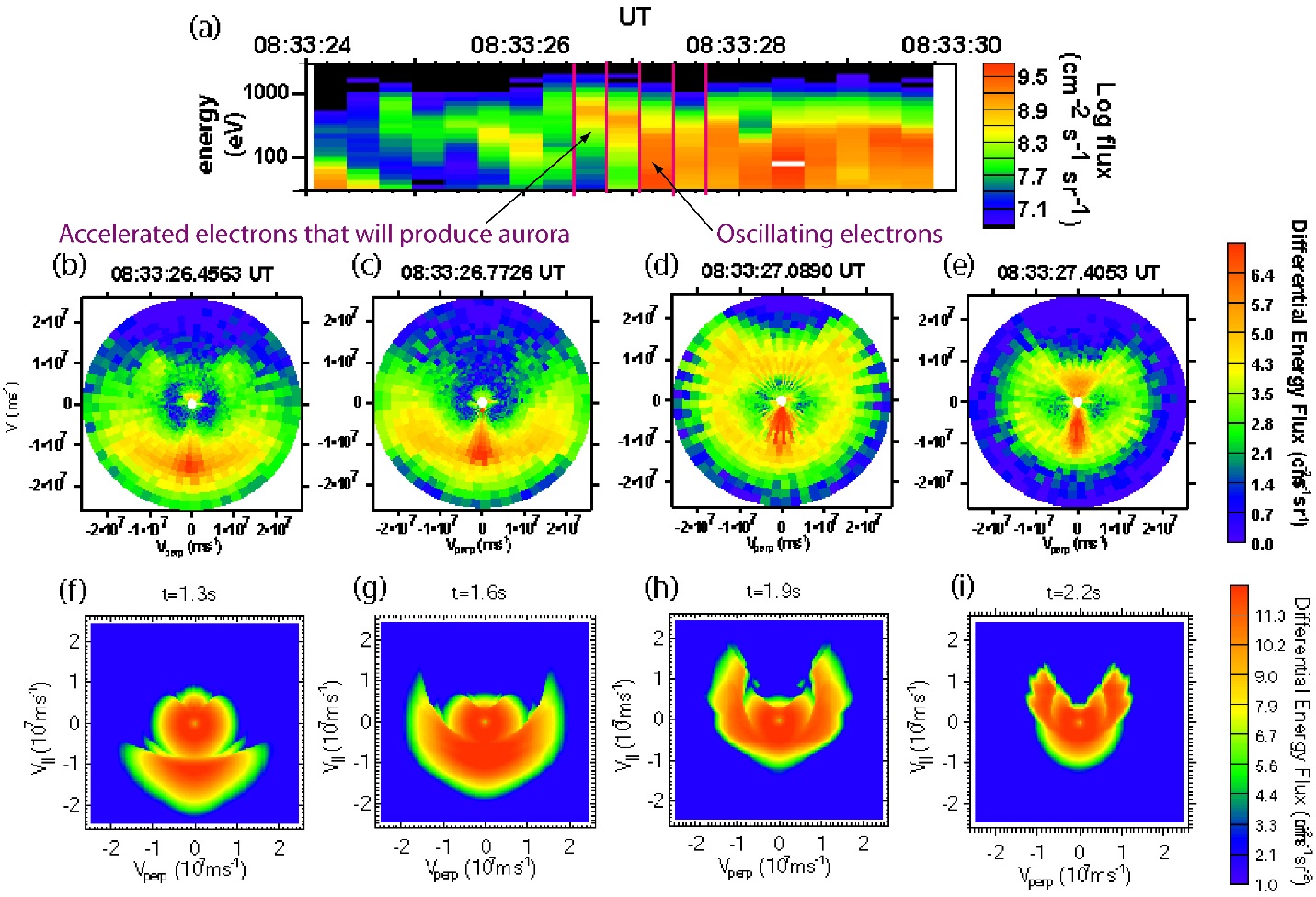
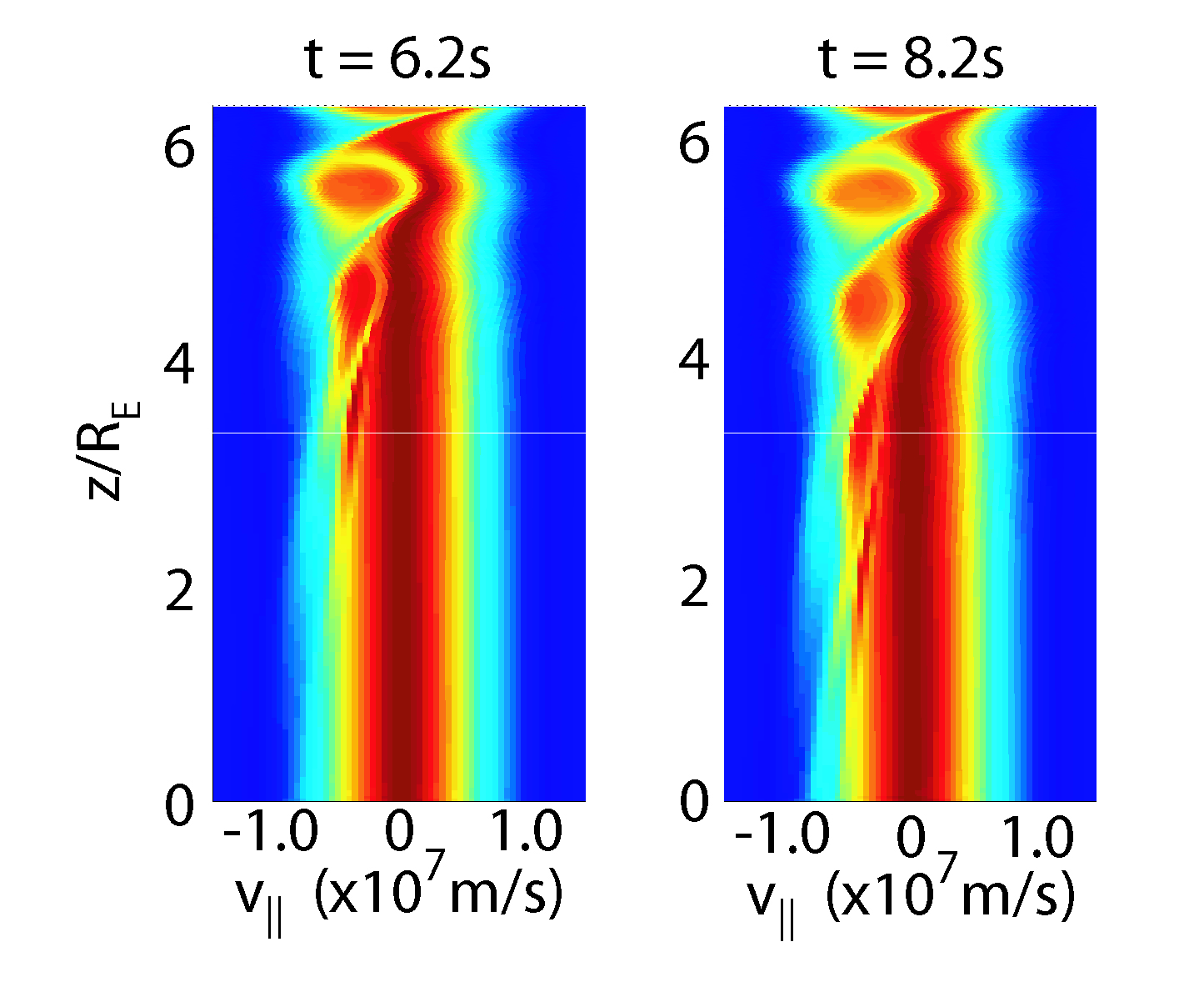
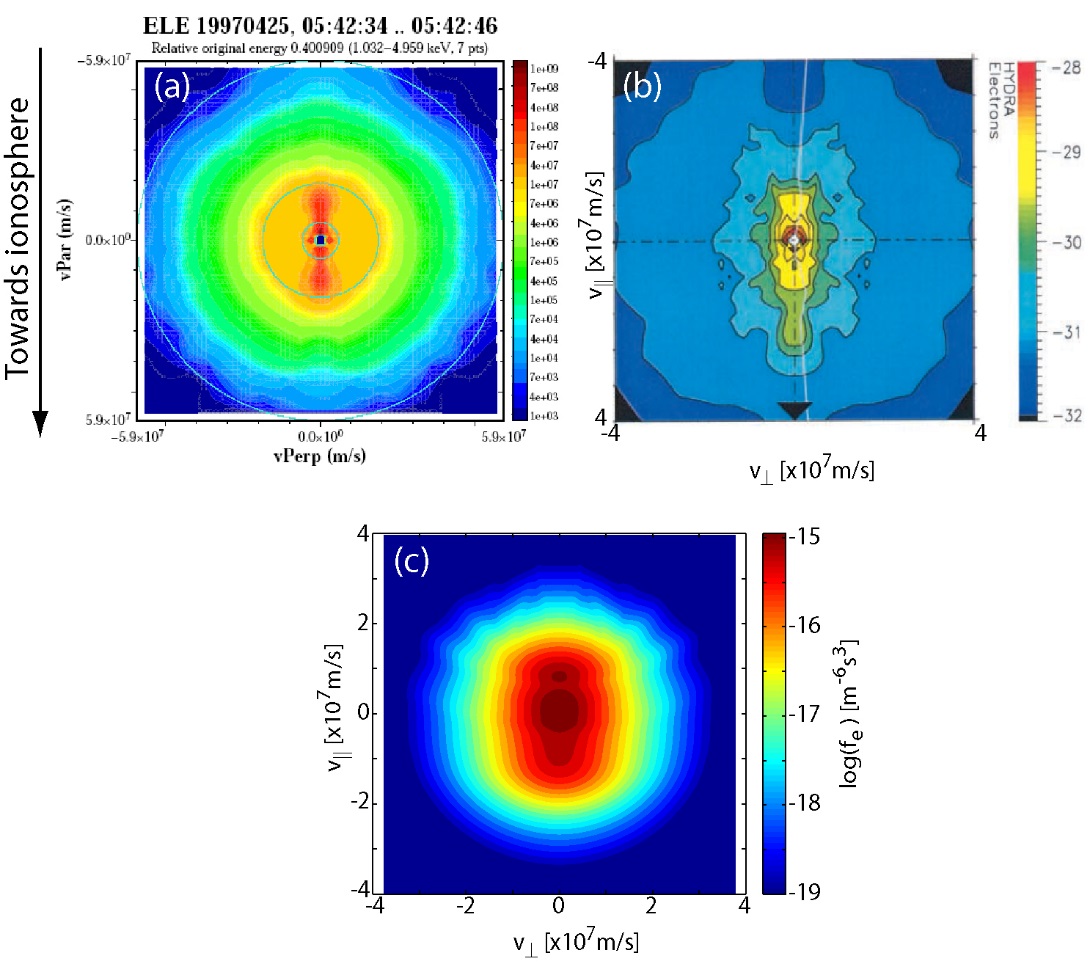